近日,《纳米快报》(Nano Letters)发表了题为Excitonic Fine Structure in Emission of Linear Carbon Chain的研究论文。西湖大学理学院国际极化激元中心的Stella Kutrovskaya研究员为第一作者和通讯作者。
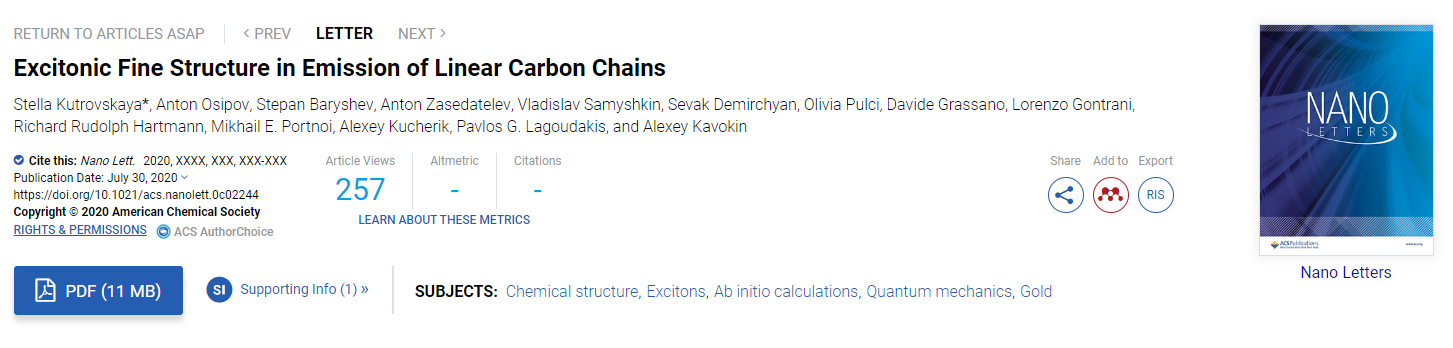
论文链接:https://pubs.acs.org/doi/10.1021/acs.nanolett.0c02244?ref=pdf
Monoatomic carbon chains and research on excitons
Titled Excitonic Fine Structure in Emission of Linear Carbon Chains, Stella Kutrovskaya’s latest research appeared recently in a high-impact peer-reviewed journal of the American Chemical Society namely the Nano Letters. Stella Kutrovskaya, a senior research scientist from International Center for Polaritonics at Westlake University, is the first and the correspondent author there.
Wonderful Carbon
Carbon is the backbone element of our planet, although it takes up less than 0.1% of the Earth’s mass. All living organisms, from microbes to mammals, all share this in common: they are made of cells which are built on the chains of carbon atoms. Wish Carbon, the sixth element in the Periodic Table, one can build up various molecules and polymers. It is indeed amazing.
In nature, carbon is found in the form of an elementary substance that is a matter consisting solely of atoms of one type of a chemical element. We know these free carbon states as diamond, graphite and coal. By forming bonds of different shapes, carbon atoms take allotropic modifications and have very different properties. For example, diamond has a three-dimensional tetrahedral structure, where each carbon atom is surrounded by for identical atoms, they form a pyramid; the chemical bonds are very strong, and diamond is therefore very hard. Graphite is another example. It is a multi-layer structure, where carbon atoms lying in one layer are firmly bound with three identical atoms into hexagons. But the distance between layers are weak, therefore the atom layers are easily peeled off, and therefore would leave marks on a piece of paper.
Since 21st century, more and more elementary carbon substance is discovered: fullerene, carbon nanotubes, graphene, etc. They exhibit unique electronic properties that make them promising for a variety of applications in nanoelectronics and photonics. Therefore, these low-dimensional crystals based on carbon were attracting the attention of the physical and chemical research communities. Monoatomic carbon chains are among them.
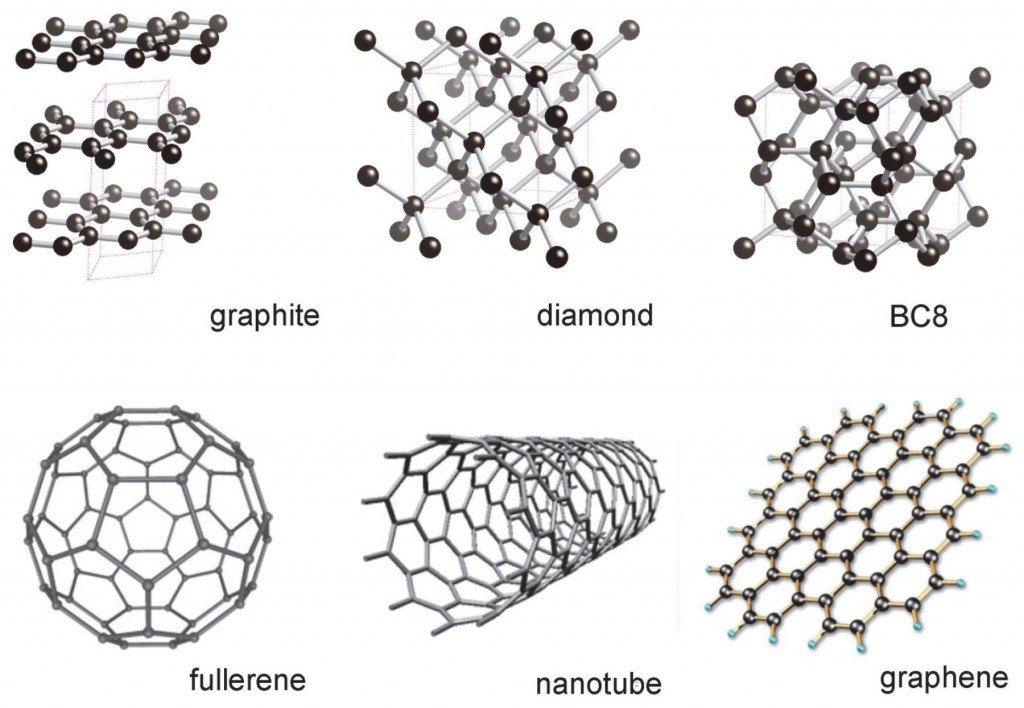
Fig.1 Various elementary substance of carbon
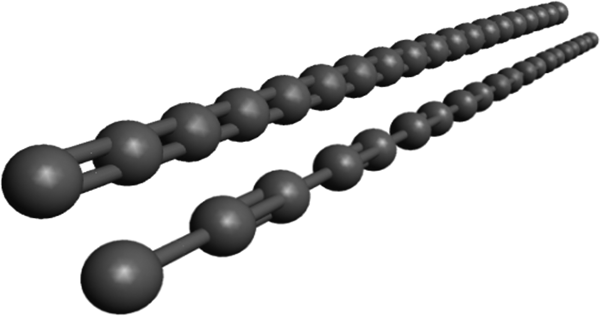
Fig.2 Two elusive allotropic forms of monoatomic carbon chains:
Cumulene (=C=C=)n (top) and polyyne (-C≡C-)n (bottom)
Unique mechanical and optical properties
Monoatomic carbon chains have two allotropic forms: cumulene and polyyne. According to recent theoretical works, one-dimensional carbon chains could form the most robust of all known crystals [1]. Polyyne, where single and triple electronic bonds alternate, the interatomic distances are predicted to be 0.133 nm(C-C)and 0.123nm (C≡C). Cumulene, where neighboring carbon atoms are linked with double electronic bonds, has an interatomic distance(0.129nm) shorter than in graphite(0.142nm). This is why the predicted Young’s modulus of linear carbon chains is somewhat higher than graphene and 1 order of magnitude higher than that of diamond.
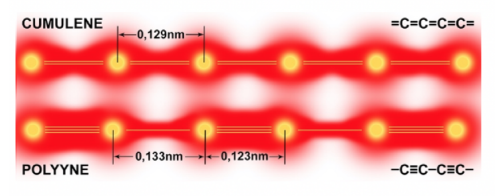
Fig.3 Schematic distribution of the electron density in cumulene and polyyne allotropes.
Calculations show that infinite-chain polyyne is a direct band gap semiconductor, and it has unusual optical properties. For example, unlike graphite or graphene which are strong absorbers and do not emit light and carbon nanotubes where the multivalley band structure leads to dark excitons suppressing the luminescenceone, one-dimensional carbon crystals can emit visible light. They have a high potentiality for optoelectronic applications, especially in nanolasing and single photon emitters.
Researchers made breakthroughs on synthesis
Yes, the monoatomic carbon chains have unique physical properties and application potentials, but how can we obtain them in the first place?
Traces of two stable allotropes of sp-carbon (polyyne and cumulene) have been found in nature: in meteorite craters, interstellar dust, natural graphite, and diamond mines. However, it is difficult to extract freestanding carbon chains from natural sources. The high chemical reactivity of linear acetylenic carbon and its low stability at room temperature and atmospheric pressure make it difficult to extract freestanding carbon chains from natural sources. Moreover, multiple attempts to synthesize polyyne chains artificially have yielded modest or no success so far. Their synthesis appears to be a formidable challenge, and many works have been devoted to the artificial stabilization of carbon chains.
Kutrovskaya and her collaborators synthesized stable polyyne chains by the laser ablation (LAL) technique in a colloidal solution. The mechanical stabilization of elongated structure was achieved due to the gold nanoparticles of different sizes attached to the two ends of the chains (Fig. 4). When deposited on a substrate, the stabilized chains demonstrated straight parts whose lengths significantly exceed the theoretical limit for a free stable monatomic carbon chain. The high-resolution transmission electron microscopy (HR TEM) shows straight linear carbon chains of the lengths that sometimes exceed 5 nm (Fig.5).
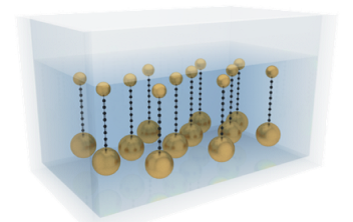
Fig.4 Concept of stabilization of monatomic carbon chains by gold nanoparticles (NPs) in a solution.
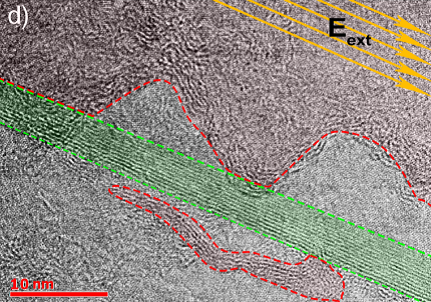
Fig.5 HR TEM image. The green area corresponds to the field-aligned polarized chains; the red area is occupied by randomly oriented unpolarized chains.
Excitonic effects of carbon chains
In the low-temperature photoluminescence (PL) measurements, researchers observed a fine structure that emerges on the top of wide picks characterising the emission of light by carbon chains of different lengths dominantly containing from 8 to 18 atoms. The triple PL structure is nearly identical in the carbon chains of a different length and it’s invariably composed of a sharp intense peak accompanied by two broader satellites shifted by about 15 and 25 meV to the lower energy side of the main peak. To associate the observed triple structure with an exciton and trion transitions, we did supplementary measurements to reveal a full set of excitonic properties of carbyne with our own ab-initio calculation. The charged exciton complexes (trions) are likely to be formed in our system due to the vicinity of metal nanoparticles that can supply carbon chains with extra charge carriers, and this is confirmed by the theoretical calculation of energy splitting with experimental data. Kutrovskaya comments: “The gold-stabilized carbon chains showed distinguished excitonic signals at low temperature, as they have a very strong spatial
localization and other features.”
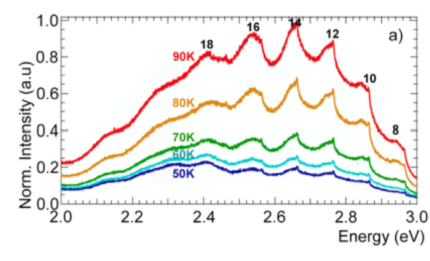
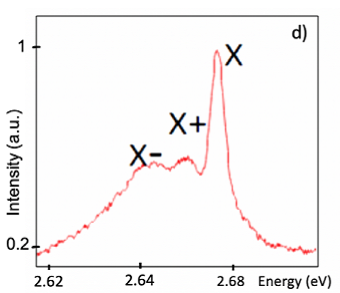
Fig.6 PL spectra of the deposited polyyne chains of different length (left). Triple PL structure shows up in spectrum at temperature 4K on a top of (right).
Kutrovskaya has been developing since 2012 the new methods for the synthesis, stabilization and deposition of ultimate one-dimensional crystals: the carbynes. “As they are instable in vacuum, their synthesis is challenging, and no group could demonstrate macroscopically long free standing carbyne crystals till now.” Kutrovskaya said, “These results shed light on the physics of one-dimensional crystals and pave the way to realization of a new set of quantum nanoelectronic and nanophotonic devices including the nanometer-size diodes and transistors, single and entangled photon emitters.”
The fundamental physics of monoatomic carbon chains is extremely rich, and the impact of this work on the overall development of the solid-state physics may be comparable with the impact of the introduction of graphene in 2004. Kutrovskaya and her colleagues are building up a lab within the International Center for Polaritonics at the Westlake university. They plan fabricating nanoelectronic networks based on linear carbon chains that will be studied with use of high precision electronic microscopy, quantum transport measurements, cryogenic time-resolved optical spectroscopy and photon correlation measurements.
[1] G. Casillas, A. Mayoral, M. Liu, A. Ponce, V.I. Artyukhov, B. I. Yakobson, and M.Jose-Yacaman, New insights into the properties and interactions of carbon chains as revealed by HR-TEM and DFT analysis, Carbon 66, 436 (2014).
[2] H. Kroto, Carbyne and other myths about carbon, Chemistry World 7 (2010).
神奇的碳
碳是我们这颗行星上的主要元素,约占了地球质量的0.1%。地球上所有的生命,从微生物到大型哺乳动物,都有一个共同点,即它们都是由细胞组成的。而细胞的基础就是碳原子链。“碳”在元素周期表里排第六,这个元素能构建出各种各样的分子和高分子,真令人不可思议。
在自然界,还存在很多形式的碳单质,它们由单一的碳原子组成的。这些处于游离态的碳,就是大家所认识的钻石、石墨、煤。是的,碳原子之间可以形成多种晶体键,碳元素以不同方式排列形成这些同素异形体,它们的性质迥然不同。比如,钻石是三维的四面体结构,每个碳原子周围是四个相同的原子,形成四面体金字塔;碳原子之间形成非常强的化学键,因此钻石特别坚硬。又比如,石墨是个多层结构,同一层中每个碳原子和其他三个碳原子紧密连接,形成正六边形;而两层之间相距较远,因此它们之间的连接很弱,正因为此,石墨制成铅笔可以拿来图画:白纸上留下的是一层层被剥落的碳原子。
21世纪以来,人们发现了更多的碳单质。富勒烯、碳纳米管、石墨烯等呈现出不同寻常的电学特性,可用于纳米电子、光电子等应用开发。于是,这些低维碳基晶体越来越被物理学和化学研究人员所重视,其中就包括单原子碳链。
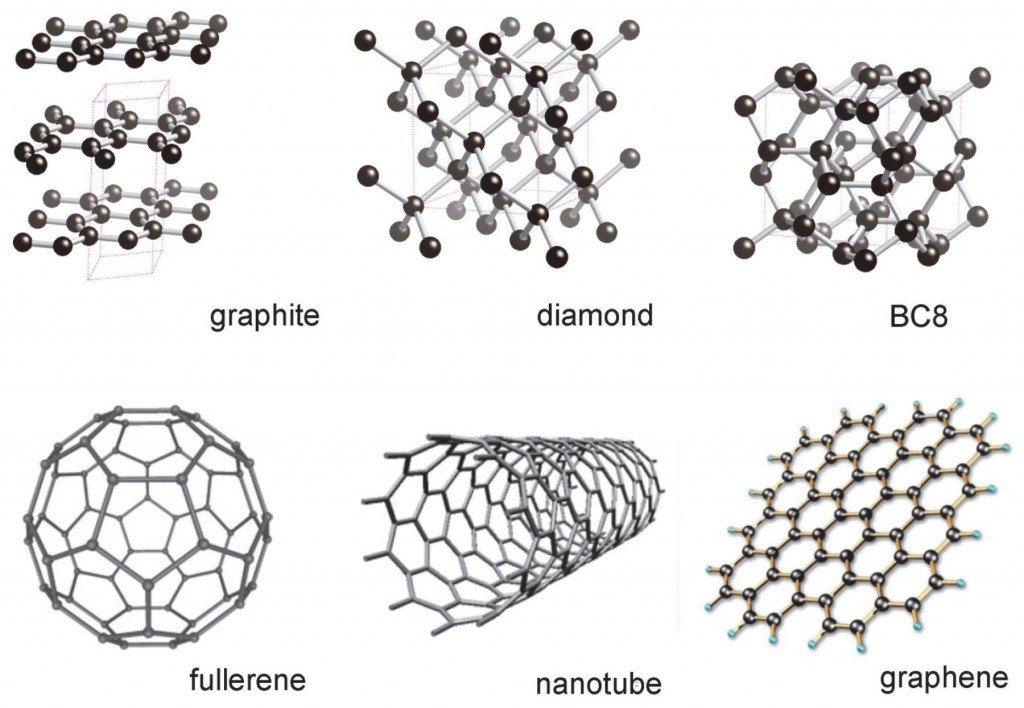
图1 各种形态的碳单质(图片来自网络)
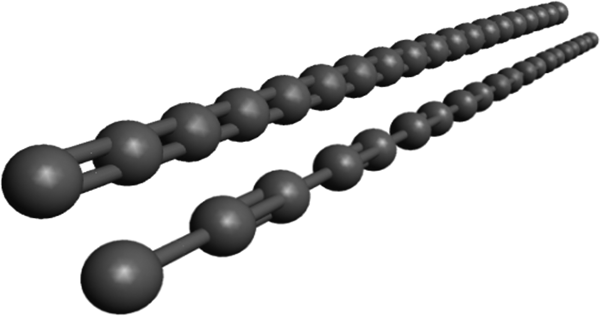
图2 单原子碳链的同素异形体:聚集二烯烃(上)和聚炔烃(下)
独特的力学和光学性质
单原子碳链有两种稳定的同素异形体:聚集二烯烃(cumulene)、聚炔烃(polyyne)。根据理论预测,这种一维碳链是世界上最坚固(robust)的晶体[1]。其中,聚炔烃中的碳原子之间是交替的一键和三键,原子间距分别为0.133nm(C-C)和0.123nm(C≡C);聚集二烯烃的碳原子之间是双键,间距0.129纳米。可见,单原子碳链的原子间距比石墨(0.142nm)要小得多。因此,碳链的杨氏模量比石墨烯高,比钻石更是要高出一个量级。
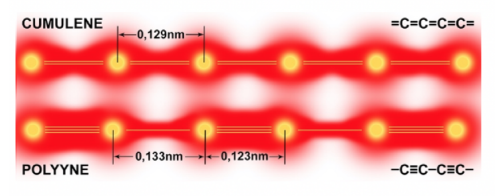
图3 聚集二烯烃的碳原子之间是双键(上);聚炔烃中的碳原子之间是交替的一键和三键(下)
根据计算,无限长的聚炔烃是一种直接带隙半导体,因而具有特殊的光学性质。比如,它能发射可见光;比起会吸收光的石墨、会抑制发光的多能谷的碳纳米管,它更适合做发光器件。又比如,它的光学非线性非常大,可用于光电子器件和激光的开发。
研究人员攻克制备难关
单原子碳链具有独特的物理特性和应用价值的,但人们如何获得单原子碳链?
在自然界,我们可以在陨石坑、星际尘埃、天然石墨和钻石矿里中找到单原子碳链的两种同素异形体,但要将它们分离出来并不容易。这是因为,在室温和大气常压下,这些线性炔键碳极易发生化学反应,非常不稳定。从自然界提取不易,人们于是又尝试了各种方法来人工合成,然而要想获取稳定的单原子碳链,到目前为止进展都不大[2]。当前的研究主要专注于如何让碳链稳定,。
Kutrovskaya与其合作者运用激光烧蚀技术在胶质溶液中合成了聚炔烃。单原子碳链的两端和金纳米粒子以电子键相连,碳链因而获得了力学上的稳定(见图4)。接下来,研究人员将这些碳键沉积到基底,进行观察和测量。通过高清电子透镜(HR TEM),研究人员发现,一些碳链在极化作用下伸直,长度可达5nm以上,超过了理论预测的极限(图5)。
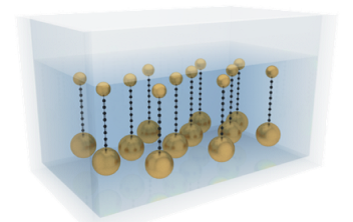
图4 用金纳米粒子稳定的单原子碳链
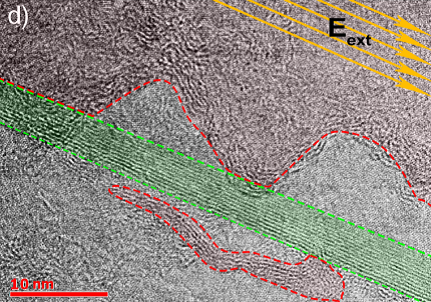
图5 高清电子透镜图。绿色区域是经极化拉直的单原子碳链,红色区域是取向随机的碳链。
碳链的激子效应
通过低温光致发光光谱表征,研究人员能清晰分辨,这些单原子碳链含原子个数为8到18个不等。而当温度降到4K时,他们更是看到了更为精细的光谱结构。结合理论分析,研究人员排除了其它可能性,并认为在低温下出现的这些峰,表明了在这些碳链中存在基于电子边缘态的激子(exciton)。而该系统中形成带电激子(trions),很可能是由于和临近的金属纳米粒子为碳链提供了额外的电荷,实验数据也符合对带电激子能量裂距的计算。Kutrovskaya说:“低温下如此显著的激子信号,可见由金纳米粒子稳定的碳链的空间局域化很强。”
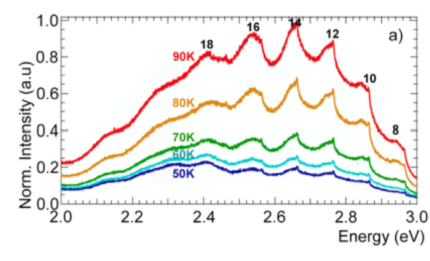
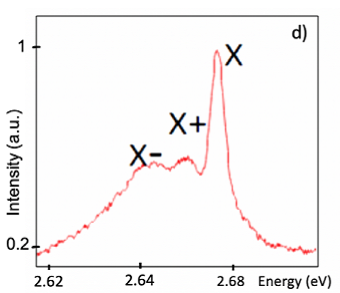
图6 沉积于基底上的单原子碳链的光致发光光谱。(左)光谱谐振对应含8到18个原子长度不等的碳链。(右)当温度降至4K,出现更为精细的光谱结构,
Kutrovskaya从2012年起就开始研究合成、稳定、沉积一维单原子碳链的新方法。“它们在真空中不稳定,所以合成工作具有相当的挑战性,至今世界上还没有团队展示过独立的长链。之前我在俄罗斯,现在我在西湖大学开展研究,用金来稳定碳链,终于成功地合成了稳定的单原子碳链。” Kutrovskaya介绍说,“这些结果,为研究一维晶体的物理性质创造了一个良好的开端。我们可以用碳链来实现全新的量子纳米器件和纳米光电器件,包括纳米尺度的晶体管、单光子发射器、纠缠光子发射器等。”
单原子碳链蕴含着极其丰富的基础物理学。类比2004年石墨烯的出现,单原子碳链也将对固体物理的整体发展发挥巨大的影响力。未来,Kutrovskaya和同事正计划搭建一个基于碳链的纳米电子网络,目前正在理学院国际极化激元中心的搭建实验室,将开展高精度电子显微测试、量子输运测试、低温光谱测试等实验研究。
[1] G. Casillas, A. Mayoral, M. Liu, A. Ponce, V.I. Artyukhov, B. I. Yakobson, and M.Jose-Yacaman, New insights into the properties and interactions of carbon chains as revealed by HR-TEM and DFT analysis, Carbon 66, 436 (2014).
[2] H. Kroto, Carbyne and other myths about carbon, Chemistry World 7 (2010).